GNSS的增量改进
Examining the Developments in Constellations, Augmentation and Receivers
It is almost impossible to imagine geoinformation without GNSS nowadays. Even most geodetic services have switched to GNSS to maintain the geodetic reference network rather than using traditional optical techniques. This article explores the state of the art of GNSS, from both a constellation, augmentation and receiver perspective. Spoiler alert: it is more of an evolution than a revolution!
Global satellite navigation systems (GNSS) are everywhere. If you try to imagine geoinformation without GNSS, you will probably fail. Some people might say: ‘But what about my total station?’. But perhaps they are forgetting that most geodetic services have long since stopped surveying the control points using traditional, optical techniques and have switched to GNSS to maintain the geodetic reference network instead. So what is the current state of play when it comes to GNSS?
GNSS Constellations
GNSS星座的数量没有改变over the past few years. Listed in the order in which they first became fully operational in their current configuration, they are: the USA’s GPS, Russia’s Glonass, China’s Beidou and Europe’s Galileo. Actually, Galileo should not be included if this is a list of fully operational systems, since – at the time of writing – only 21 of the planned 27 plus 3 satellites were fully usable, with another two in the commissioning phase. Hopefully, Galileo will also reach its full operational status at some point in 2022.
Besides these four global navigation satellite systems, there are two regional systems. The Japanese QZSS is probably the best-known one; with both geostationary and quasi-zenithal geosynchronous satellites, the system provides coverage of Japan. Another three satellites are planned for QZSS in 2023, bringing the constellation to seven satellites which will provide positioning over Japan (and Australia). The second regional system is NavIC (or IRNSS, as it was known until 2016), which orbits over India. Again with both geostationary and geosynchronous satellites, this system covers India and the Indian Ocean. As both QZSS and NavIC are purely regional, they cannot be used outside their particular region. This is in stark contrast to the ‘true’ GNSS, although Beidou is actually both regional and global; the system employs geostationary and geosynchronous satellites over China, as well as satellites that orbit the world in a medium Earth orbit like the other three GNSS. The advantage of this is greatly improved coverage over China (and Australia). As a result, the people with the best GNSS coverage in the world are probably the Australians, with a total of five available systems. India can also use five systems for navigation, although it is closer to the edge of Beidou’s coverage.
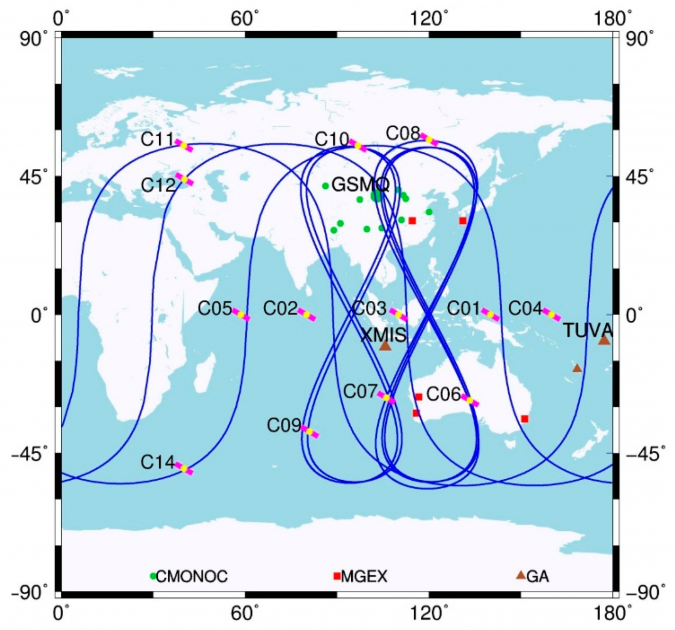
In terms of development within the various systems, the signal structure has remained the same for most systems and improvements have mainly been made in the control and positioning of the satellites themselves. Although relatively small improvements, they do have a sizable impact because a more accurately positioned satellite translates into better on-the-ground accuracy. The only system itself that has been improved is Glonass. Starting in 2018, a series of satellites were launched with the new L3OC signal. This CDMA signal is easier to implement than the so-called ‘standard’ FDMA signals because the CDMA signal uses similar techniques to those in the other GNSS. However, it has now been well over a year since the last launch (in 2020) and – especially considering the current conflict situation – the current plans for Glonass are unclear.
GNSS Augmentation
无论是用于测量和其他专业地理信息工作,智能手机还是在车辆上,以及在美国,欧洲或亚洲,它很少是使用的“原始” GNSS信号,而是改进的,增强的信号。这要归功于许多“自由”(即政府赞助的)空间基础增强系统(SBAS):美国的WAA,日本的MSA,欧洲的EGNOS和印度的Gagan。其他系统处于开发或认证的各个阶段,包括SDCM(俄罗斯),卡斯(韩国),BDSBAS(中国),南潘(Southpan)(澳大利亚),SACCSA(南/中美洲和加勒比海)和A-SBA(赤道非洲)。这些系统基本上以相同的方式发挥作用。地面上的控制站(“基本”)接收卫星定位信号,并将实际收到的信号与预期的信号进行比较。基于这些测量值,开发并将一组校正开发并传输到地静止卫星,从那里将它们发送回GNSS频带中的接收器。这些卫星通常可以通过它们显示的“高”卫星数字在天空中识别。
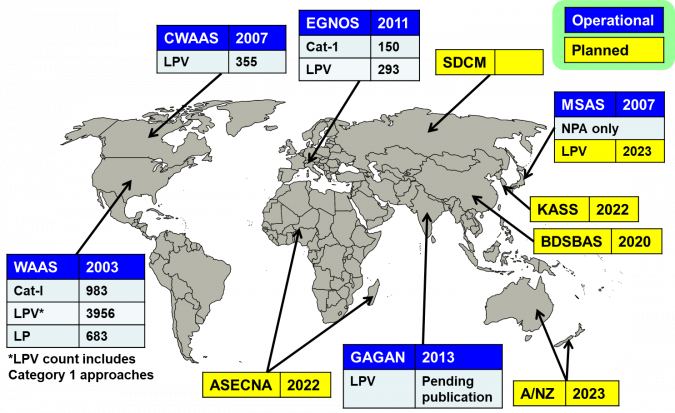
由于SBA覆盖的面积相当大,因此最终的准确性是合理的,但不是很高。当土地调查中需要高的水平和垂直精度时,使用实时运动学或RTK扩展系统。根据设置的不同,测量师可以使用自己的基本站进行UHF校正,或者使用现有网络。如果使用了现有网络,他们可以使用单个基础的更正 - 但是通常,他们使用虚拟参考站,网络“计算”一组校正,就好像基站位于调查区域或附近一样。当使用自己的基站并进行后处理(而不是实时使用它们)时,甚至可以实现次级中心精度。RTK的缺点是该范围限于距(虚拟)基站约15公里。因此,RTK的使用可能是有问题的,尤其是在有限的大地测量或通信基础架构的偏远地区。
Precise point positioning (PPP) lies somewhere in between these two technologies. Although it is not as accurate as RTK, the advantage of PPP is that it works over large areas without having to install one’s own base station. In PPP, data from ground-based stations is used to model the local errors in the GNSS observations (rather than transmitting corrections). Using an iterative process of predicting the model errors and comparing them to the real results, the receiver iterates stand-alone positioning to a high accuracy. A data link is still required in order to receive the model data, but this method is not limited by a range of just a few kilometres or the need to have local reference points. The latest commercial services using all available GNSS coverage are now achieving sub-decimetre results in terms of both horizontal and vertical precision.
伽利略高精度服务基于相同的原理,但水平精度约为20厘米,垂直精度约为40厘米。该服务预计将在2022年最初运营,并从2024年开始全面运营。对于单点,通常可以通过各种自由使用的后处理服务(例如AUSPOS CSRS-PPP和Trimble Centerpoint RTX后处理后处理)获得与商业PPP系统类似的结果。根据服务的不同,甚至可以使用后处理运动数据,而不仅仅是静态数据。
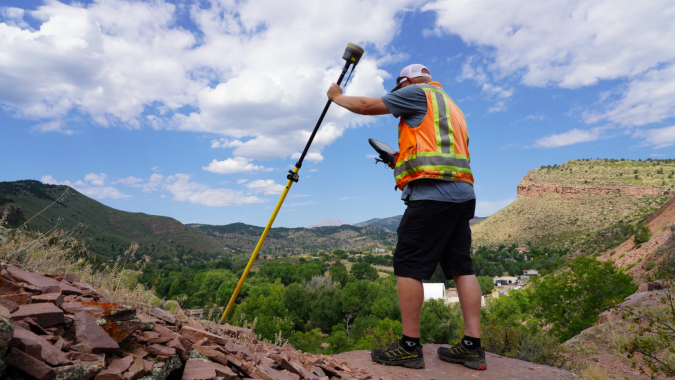
GNSS Receivers
Just as with all hardware, the GNSS receiver is continuously being further developed. However, based on recent specifications, it is apparent that the development is more of an evolution than a revolution. Perhaps the greatest change to the GNSS receiver has been the addition of an IMU, allowing the user to hold the pole ‘at an angle’ while measuring. Although this introduces an additional error from the IMU, it allows the user to conduct surveys in difficult-to-reach places. Moreover, if the utmost accuracy is not required, surveys can be completed much faster too since the user no longer needs to wait for the bubble to centre before taking a measurement. Depending on the brand and model of the receiver, the achievable angles are anywhere between 15° and 60° of tilt.
Needless to say, the second major development is that the units are becoming ever-smaller for use on unmanned aerial vehicles (UAVs or ‘drones’). Whereas post-processed kinematic on a drone would have been regarded as a benefit a few years ago, nowadays multi-frequency RTK systems are starting to become the standard for high-end systems. This trend is enabling surveyors to obtain much better models without requiring as many ground control points to tie the photographs into the geodetic network.
Conclusion
As outlined above, there have been incremental changes in GNSS positioning over the last few years. With ever-growing GNSS constellations, further developments in augmentation systems and upgrades to receivers, things seem set to continue to improve in the (near) future.

使您的收件箱更有趣。Add some geo.
Keep abreast of news, developments and technological advancement in the geomatics industry.
免费注册